A team of Princeton University physicists has achieved a breakthrough in quantum mechanics by entangling single molecules. This research opens up new possibilities for quantum computing, simulation and sensing. The team’s innovative use of optical tweezers to control molecules overcame previous challenges in quantum entanglement and marked a major advance in the field. Image source: SciTechDaily.com
In work that could lead to more robust Quantum computingPrinceton University researchers have successfully forced molecules into quantum entanglement.
For the first time, a team of Princeton University physicists has been able to link single molecules into a special state of quantum mechanical “entanglement.” In these strange states, molecules are still related to each other and can interact simultaneously, even if they are miles apart, or, indeed, even if they occupy opposite ends of the universe.The research was published in the journal science.
Molecular Entanglement: A Breakthrough for Practical Applications
“This is a breakthrough in the molecular world because of the fundamental importance of quantum entanglement,” said Lawrence Cheuk, assistant professor of physics. Princeton University and senior author of the paper. “But it’s also a breakthrough for practical applications, as entangled molecules could form the basis for many future applications.”
These include, for example, quantum computers that can solve certain problems faster than traditional computers, quantum simulators that can model complex materials whose behavior is difficult to model, and quantum sensors that can measure faster than traditional sensors. .
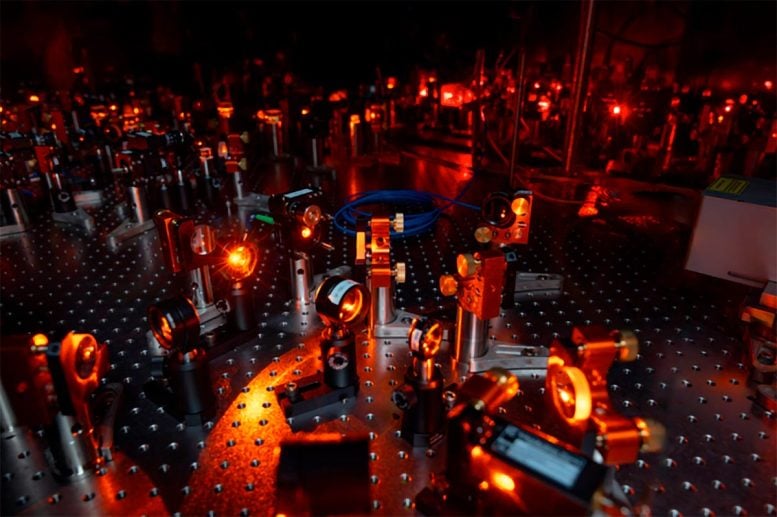
Laser device used to cool, control and entangle single molecules.Image credit: Richard Soden, Department of Physics, Princeton University
“One of the motivations for doing quantum science is that in the real world, it turns out that if you exploit the laws of quantum mechanics, you can do better in many fields,” said Connor Holland, a graduate student in the Department of Physics and author of the work. Co-author.
The ability of quantum devices to surpass classical devices is called “quantum advantage.” At the heart of quantum advantage are the principles of superposition and quantum entanglement. While classical computer bits can assume the value of 0 or 1, quantum bits (called qubits) can be in a superposition of 0 and 1 at the same time. The latter concept, entanglement, is a major cornerstone of quantum mechanics and occurs when two qubits are present at the same time. The particles become inseparable from each other, so that the connection remains even if one particle is light-years away from another. Albert Einstein, who initially questioned its validity, described the phenomenon as “spooky action at a distance.” Since then, physicists have shown that entanglement is actually an accurate description of the physical world and how reality is constructed.
Challenges and Progress of Quantum Entanglement
“Quantum entanglement is a fundamental concept,” said Zucker, “but it’s also a key factor in giving quantum advantage.”
But establishing quantum advantage and achieving controllable quantum entanglement remains a challenge, not least because engineers and scientists still don’t know which physical platform is best suited for creating qubits. Over the past few decades, many different technologies—such as trapping ions, photons, superconducting circuits, and more—have been explored as candidates for quantum computers and devices. The optimal quantum system or qubit platform will likely depend on the specific application.
However, until this experiment, molecules had long been unable to achieve controllable quantum entanglement. But Zucker and his colleagues found a way to control single molecules and coax them into these interlocked quantum states through careful manipulations in the laboratory. They also believe that molecules have certain advantages (over atoms, for example) that make them particularly suitable for certain applications in quantum information processing and quantum simulations of complex materials. For example, molecules have more quantum degrees of freedom than atoms and can interact in new ways.
“In practical terms, this means there are new ways to store and process quantum information,” said Yukai Lu, a co-author on the paper and a graduate student in electrical and computer engineering. “For example, a molecule can vibrate and rotate in multiple modes. So you can use two of those modes to encode a qubit. If the molecule kind Being polar, two molecules can interact even when spatially separated. “
Still, it proved difficult to control in the laboratory due to the complexity of the molecule. Their freedom makes them attractive, but also makes them difficult to control or keep in captivity in laboratory settings.
Innovative Experimental Technology and Future Prospects
Cheuk and his team addressed many of these challenges through thoughtful experiments. They first selected a molecular species that was both polar and could be cooled with a laser. They then laser-cooled the molecules to ultracold temperatures where quantum mechanics takes center stage. Single molecules are then picked up by a complex system of tightly focused laser beams, so-called “optical tweezers.” By engineering the position of the tweezers, they were able to create large arrays of single molecules and position them individually into any desired one-dimensional configuration. For example, they created isolated pairs of molecules and defect-free strings of molecules.
Next, they encoded the qubits into the non-rotating and rotating states of the molecule. They were able to show that this molecular qubit remained coherent, that is, it remembered its superposition state. In short, the researchers demonstrated the ability to create well-controlled and coherent qubits from individually controlled molecules.
In order for molecules to become entangled, they have to make the molecules interact with each other. By using a series of microwave pulses, they were able to make single molecules interact in a coherent manner. By allowing precise timing of interactions, they were able to achieve a two-qubit gate that entangles two molecules. This is important because such entangled two-qubit gates are building blocks for universal digital quantum computing and simulations of complex materials.
Given the innovative capabilities offered by this new molecular tweezer array platform, the potential of this research to investigate different areas of quantum science is huge. The Princeton team is particularly interested in exploring the physics of many interacting molecules, which can be used to simulate quantum many-body systems in which interesting emergent behaviors can emerge, such as new forms of magnetism.
“Using molecules for quantum science is a new frontier, and our demonstration of on-demand entanglement is a critical step in proving that molecules can be used as viable platforms for quantum science,” said Zucker.
In another article published in the same issue scienceAn independent research team led by John Doyle and Kang-Kuen Ni of Harvard University and Wolfgang Ketterle of MIT achieved similar results.
“The fact that they got the same results validates the reliability of our results,” Zucker said. “They also show that molecular tweezer arrays are emerging as an exciting new platform for quantum science.”
Reference: “On-demand entanglement of molecules in reconfigurable optical tweezer arrays” by Connor M. Holland, Yukai Lu and Lawrence W. Cheuk, December 7, 2023 science.
DOI: 10.1126/science.adf4272
This work was supported by Princeton University, the National Science Foundation (Grant No. 2207518), and the Sloan Foundation (Grant No. FG-2022-19104).
#Quantum #Leap #Princeton #Physicists #Successfully #Entangle #Single #Molecule #Time
Image Source : scitechdaily.com